High-energy physics
Energy is key when searching for new elementary particles
High-energy physics hunts down tiny elementary particles in gigantic accelerators – and provides important insights for our understanding of the universe. But how does that work, and why do we need more collision energy for new insights?
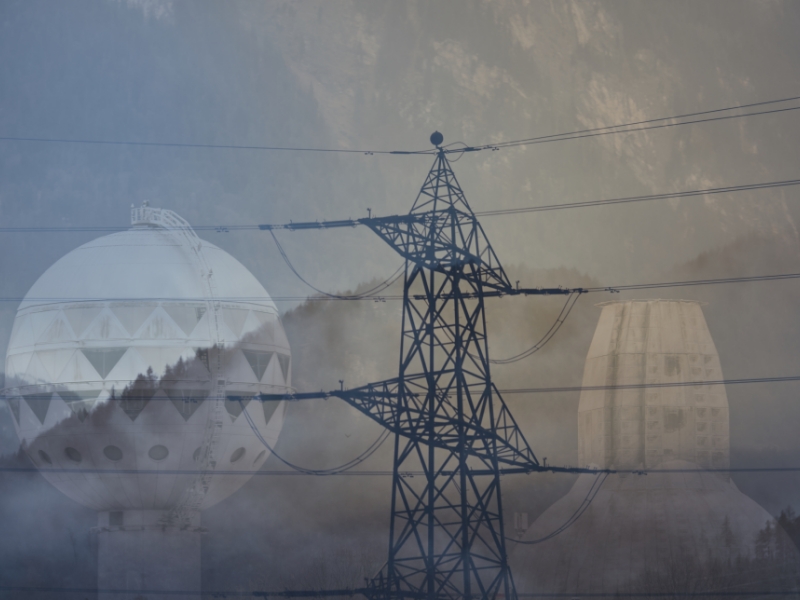
High-energy physics is actually the physics of tiny amounts of energy. At least, this seems to be the case if you take a closer look at the most important unit of measurement for energy in high-energy physics, the electron volt. If you compare it with a more common (obsolete) unit of energy in everyday life, the calorie, you will see that a single calorie corresponds to about 26 quintillion electron volts (that’s a number with 18 zeros).
Of course, this comparison is flawed. But it makes one thing clear: High-energy physics is all about the tiny, microscopic world. Applied to a small building block of matter, it is possible to achieve great things with “little” energy. But how exactly does it work?
On the trail of elementary particles
High-energy physics or particle physics deals with the fundamental building blocks of matter and their interactions that determine the structure of our universe. These smallest building blocks, the elementary particles, make up the nuclei and electrons in the atoms. Atoms together form molecules, which in turn together form cells and so on.
All the knowledge about these elementary particles and the forces acting between them is summarized in what is referred to as the standard model of particle physics – the “world formula” of the smallest building blocks of matter. The last big fuss about the standard model came in 2012, when the European Organization for Nuclear Research (CERN) in Geneva announced the discovery of the Higgs boson. The sensational detection of a new elementary particle closed a large gap in the standard model of particle physics.
Subscribe to the uniAKTUELL newsletter
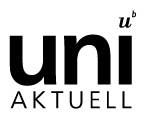
Discover stories about the research at the University of Bern and the people behind it.
But as reliably and precisely as the standard model describes everything that surrounds us, it is also clear that it is unfortunately incomplete. In the world of particle physics, there are still major questions that have not yet been answered: What exactly is dark matter? Why didn’t matter and antimatter wipe each other out again after the Big Bang?
The standard model does not (yet) have an answer to these questions. Therefore, high-energy physics continues to research tirelessly, looking for new particles and forces that could provide answers to these questions. They could fill the gaps in the standard model or even open up a completely new, previously unknown kind of physics.
How does E=mc2 work?
To find new elementary particles, you first need a particle accelerator. In these giant apparatuses, such as the Large Hadron Collider (LHC) at CERN, already known particles are accelerated to almost the speed of light and shot at each other in opposite directions. The collision produces new particles, which can then be detected and examined with particle detectors. This is what happened when the Higgs particle was discovered: It is produced during particle collisions in the LHC and was detected using the ATLAS particle detector (see box).
But why do such collisions give rise to completely new particles? To understand this, let’s take a look at Einstein and another physicist. Einstein’s famous formula E = mc2 simply states that energy and mass can be converted into one another. “This is exactly what happens in a collision in the particle accelerator,” explains Michele Weber, Professor of Particle Physics at the University of Bern. The elementary particles have only their mass as energy when at rest. “If they are now accelerated with a very high force, there is an enormous amount of acceleration energy in addition to this ‘rest energy’. On impact, this is suddenly released, resulting in completely new particles whose mass depends on the amount of energy released,” explains Weber.
“On impact, energy is suddenly released, resulting in completely new particles.”
Michele Weber
In order to understand the incredible acceleration energy required to generate new particles, let’s take a look at an example once more using electron volts. A proton (a subatomic particle) has a so-called idle energy of 938 million electron volts. In the LHC accelerator at CERN, each proton is accelerated to a maximum energy of seven trillion electron volts (7 tera electron volts TeV). Anyone who is good at calculating orders of magnitude will see that this is equivalent to 7,500 times its rest energy! The collision energy of two protons then adds up to 14 trillion electron volts, which, converted into mass, can form new particles.
More energy = new particles?
As Michele Weber puts it, the LHC is a “discovery machine”. This means that, due to the technical properties of the LHC, various possible results occur in the event of a collision, which cannot be precisely predicted in advance. This is useful in the search for new particles and worked well in the case of the Higgs boson. However, since a wide range of different particles are produced, the LHC is not well suited for the targeted study of the Higgs boson. In the opinion of many physicists, however, a closer examination of specifically this particle offers the best chance of uncovering inconsistencies in the standard model.
The fact that there were no further spectacular discoveries at the LHC after the Higgs boson is also due to the energy. With the 14 tera electron volts (TeV), the maximum collision energy that can be technically achieved with the LHC is exhausted. That’s all we can do. Therefore, the LHC cannot directly produce particles that are heavier than the mass that can be produced in a collision with these 14 TeVs. However, as the standard model is still incomplete, the conclusion is that the missing particles must either interact very weakly with other particles, which would require a higher sensitivity of the detectors. Or they are heavier and therefore to be found in the higher energy range.
The Future Circular Collider
In order to advance into higher energy spheres, a feasibility study for a new, larger particle accelerator has already been carried out. Under the name “Future Circular Collider” (FCC), CERN wants to build an accelerator that will far outshine the LHC. At the heart of the enormous structure is to be a ring tunnel with a circumference of 90 kilometers 200 meters below the Earth’s surface. By way of comparison: The LHC has a circumference of 27 kilometers. Most of the tunnel is to run under French soil, a smaller section under Swiss soil, and some even beneath Lake Geneva.
About the person
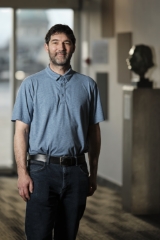
Michele Weber
is Professor of Experimental Particle Physics and Director of the Laboratory for High Energy Physics (LHEP). The Laboratory for High Energy Physics (LHEP) is a division of the Physics Institute at the University of Bern in Switzerland and is part of the Albert Einstein Center for Fundamental Physics at the University of Bern.
Two particle accelerators would be installed in the tunnel one after the other to solve several of the problems mentioned. First, an accelerator that produces precisely specific masses. In particular, the Higgs boson and other lighter and weakly interacting particles that can be studied precisely in large numbers. This is followed by a particle accelerator as a new discovery machine that builds on the infrastructure of the first and provides much greater energy than the LHC. The collision energy would be increased from the 14 TeV with the LHC to 100 TeV in order to find significantly heavier new particles. The first accelerator could start operating in the mid-2040s.
Basic research: Investing in the future
Of course, there are also criticisms of the project. The high costs, estimated at around 15 billion Swiss francs, and the enormous electricity consumption are one issue. Is this investment worthwhile in times of climate crisis? Doubts exist not only among the general public, but also among physicists. After all, there is no guarantee that you will actually find new particles with the FCC.
“I think it’s important for us to ask ourselves such questions in basic research,” says Michele Weber. As a member of the umbrella organization of Swiss particle physics researchers, he is committed to the FCC and coordinates the interests of Swiss universities in the project. You could compare the current situation in particle physics with memory game: “We have a lot of cards in front of us and don’t know what is on the reverse. I’ll never win the game if I don’t reveal the unknown cards and, in doing so, find pairs that don’t belong together. Is that a reason to stop?”
For Michele Weber, the answer is clear. Although particle physics is not necessarily trusted, its work is very interdisciplinary: “For the FCC feasibility study, it was not only physical and technical issues that had to be clarified, but also environmental and social aspects.” Such difficult research projects require innovative solutions and alternative ideas in order to achieve their goals. “We are approaching the project as responsibly as possible and believe it makes sense.”
The construction of the LHC has already shown in the past that the key to completing the standard model lies in the next highest energy order. “Energy is the key,” says Michele Weber. It brings us a little closer to the Big Bang and the formation of the universe.
High-energy physics
Cutting-edge research in Bern
Cutting-edge research in the field of particle physics is carried out in Bern. The University of Bern was a founding member of the ATLAS detector in CERN’s Large Hadron Collider. When the Higgs particle was discovered in 2012, Bernese researchers from the Albert Einstein Center (AEC) and the Laboratory for High-Energy Physics (LHEP) made significant contributions to the selection of collision events, their recording and analysis. The discovery of the Higgs boson was awarded the Nobel Prize in Physics in 2013.
Magazine uniFOKUS
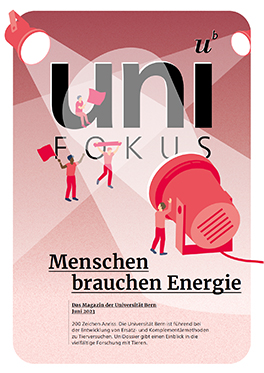
People need energy
This article first appeared in uniFOKUS, the University of Bern print magazine. Four times a year, uniFOKUS focuses on one specialist area from different points of view. Current focus topic: Energy
Subscribe to uniFOKUS magazine